What is Radiation-induced nuclear foci?
In traditional textbooks, only nucleolus had been introduced as a component of a nucleus, with the explanation that heterochromatin was the high density chromosomal DNA area, while euchromatin was the low one. However, recent progress in the technology to visualize DNA and proteins has revealed more well-organized structures in cell nucleus than expected. DNA forms chromosome in M phase for the safe cell division by preventing intermingling of each chromosomal DNA. During interphase, between M phases, chromosomal DNA is decondensed, but not mixed up in the spherical cell nuclei. Chromosomes are compartmentalized in discrete territories called chromosome territories. Various nuclear proteins also form higher order nuclear structures, named nuclear domains or foci. These higher order nuclear architectures are thought to be associated with the "fields" of DNA metabolism, replication, transcription and repair.
In irradiated cells, various proteins involved in DNA repair accumulate at the damaged site and form higher-order structures called "radiation-induced nuclear foci."
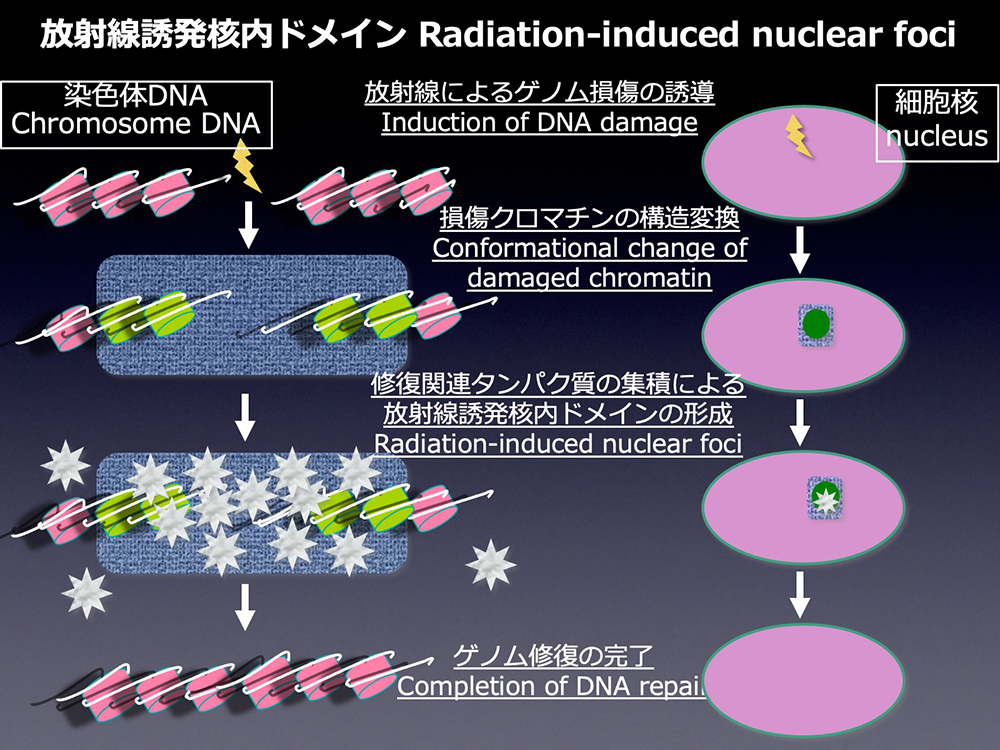
RAD51 is involved in homologous recombination repair of DNA double-strand breaks, and it forms the radiation-induced nuclear foci at the sites of DNA damage. To clarify the repair mechanism of DNA double-strand breaks, the most serious radiation-induced DNA damage, we have studied the molecular mechanism of RAD51 focus formation.
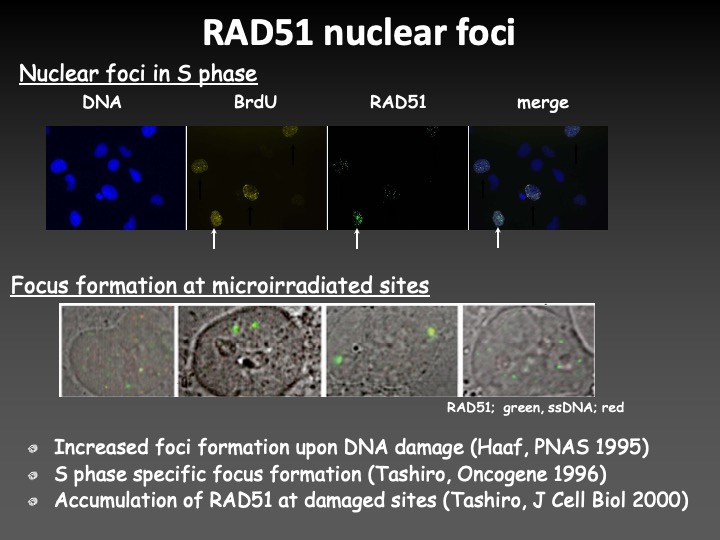
As a result, we found that the radiation-induced RAD51 focus formation required the chemical modifications and exchanges of histone variant H2AX and H2AZ-2, Lamin B1 which was known as a component of nuclear lamina, and proteins related to the nucleo-cytoplasmic shuttling systems. We also showed that interaction of RAD51 with SUMOylated proteins played a role in the radiation-induced focus formation of the protein.
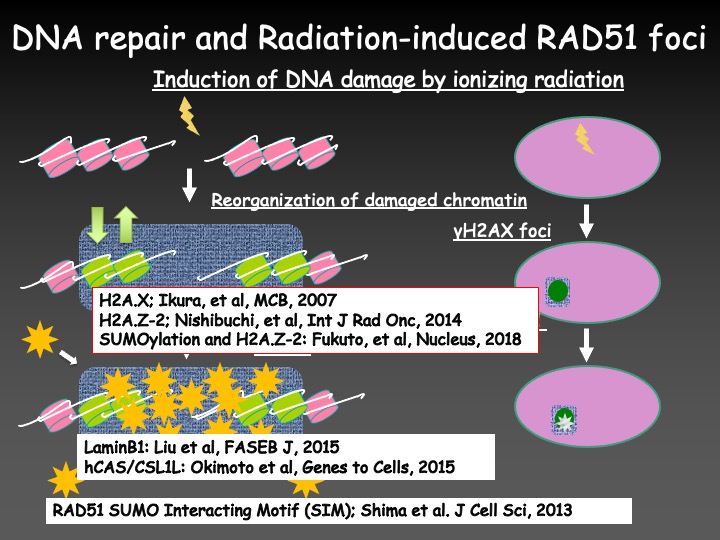
From these findings, we think that the radiation-induced nuclear domains such as RAD51 foci, are deeply involved in chromosomal aberrations by the spatiotemporal regulation of DNA repair. Therefore, we are studying the mechanism controlling the localization and dynamics of higher-order nuclear structures associated with DNA damage using the latest bioimaging technology. We are going to establish a new medical system to protect human genome from DNA damage by radiation injury. Such a medical system should be also available to apply for cancer diagnosis and therapy.
Molecular mechanisms to prevent chromosome abnormalities induced by ionizing radiation or anti-cancer drugs
DNA damages induced by ionizing radiation or anti-cancer drugs are repaired through the DNA double-strand break repair mechanism in the cell; however, if a DNA is connected to a wrong one during the repair process, structural abnormalities of chromosomes such as chromosomal translocation are formed. How do cells prevent such structural aberrations of chromosomes?
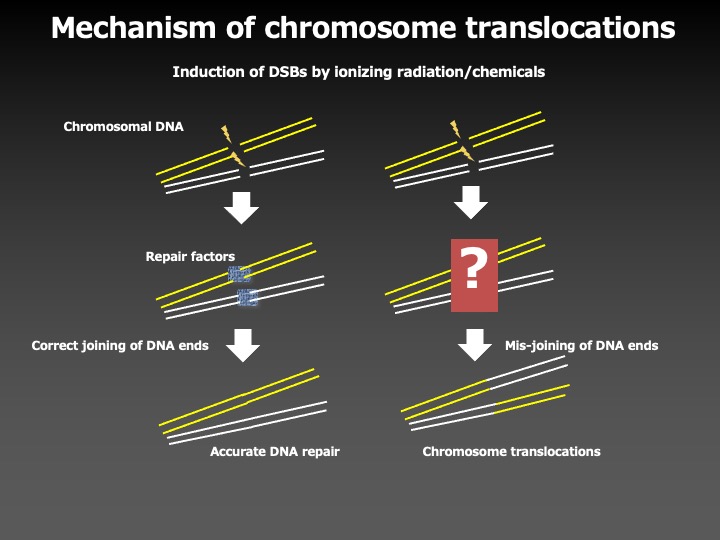
To answer this question, we investigated the molecular mechanisms to prevent chromosome aberrations, by using the 11q23 chromosome translocations, the most frequent chromosome abnormalities in secondary leukemia associated with the chemotherapy with an anti-cancer drug etoposide, as a model system.
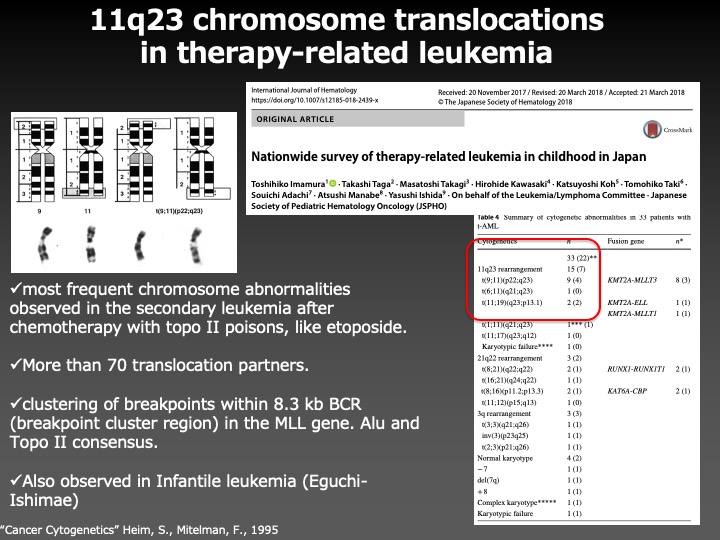
As a result, we found that the phosphorylation of ARP8, a component of the INO80 chromatin remodeling complex, by ATM, a DNA damage signaling kinase, negatively regulated the loading of chromatin remodeling complex onto the breakpoint cluster region to prevent the overloading of DNA repair factors. This finding suggests that ATM not only facilitates but also represses the DNA repair activity to adjust the loading of repair factors around DNA damage in the appropriate ranges to prevent chromosome translocations.
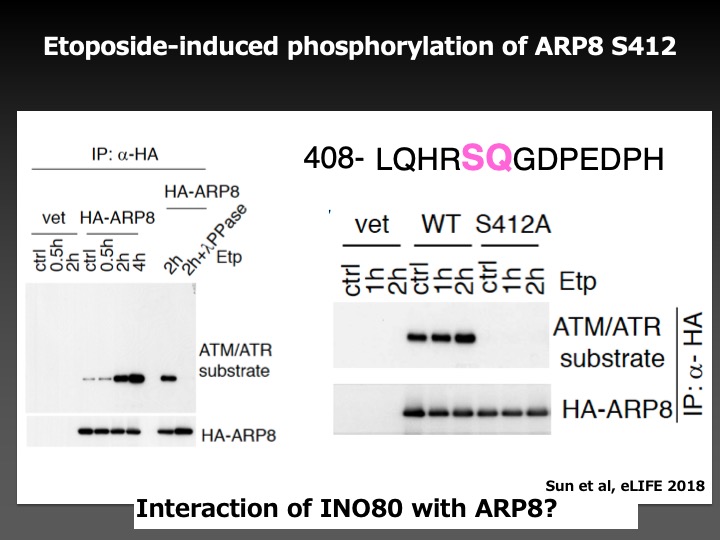
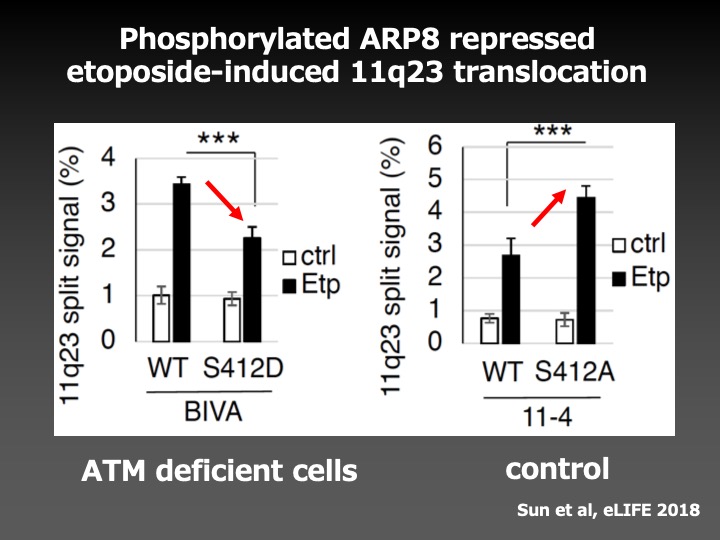
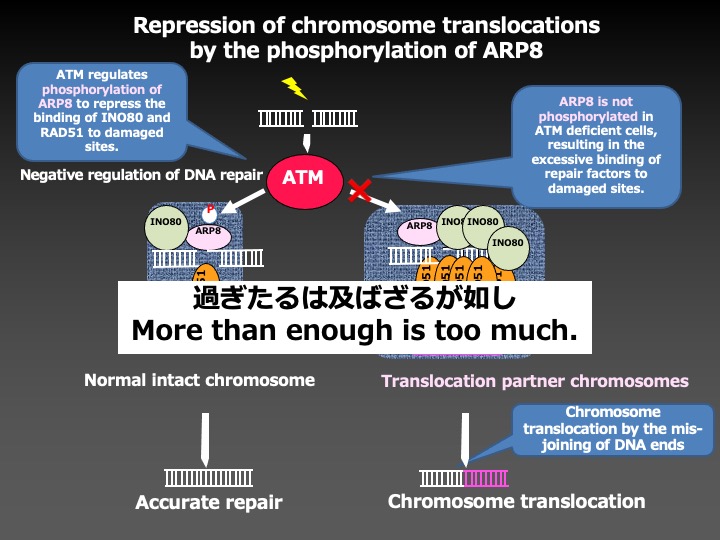
This study has revealed the important molecular mechanisms for accurate DNA repair, and will lead us to the establishment of the methods to prevent secondary malignancies associated with radiation therapy and chemotherapy.
For the development of next-generation radiology
In modern medicine, the use of radiation is indispensable for the diagnosis of diseases. Especially, the information obtained from CT examinations is very useful for screening, diagnosis, and follow-up. The safety of CT examinations, however, has been discussed because radiation induces chromosomal DNA damage and changes genetic information, leading to various health disorders such as cancer.
The health effects of radiation exposure of higher than 100 mSv have been shown by the epidemiological studies including the health surveys of atomic bomb survivors. On the other hand, those of low-dose radiation, including CT examinations with the radiation dose about 10 mSv, remained to be clarified. Since the health effects by low dose irradiation is quite small, huge epidemiological studies are required to examine such small effects.
Therefore, we decided to examine the biological effects of low dose irradiation by establishing a method to detect chromosomal abnormalities with high sensitivity. Giemsa staining is the most established method for the conventional chromosome analysis; however, the detection of abnormal chromosomes with Giemsa-stained samples requires a well-trained and experienced person. By applying the Fluorescence in situ Hybridization method using PNA probes to visualize middle and ends of the chromosomes in red and green respectively, we developed the PNA-FISH method that enabled us to analyze chromosomes more efficiently.
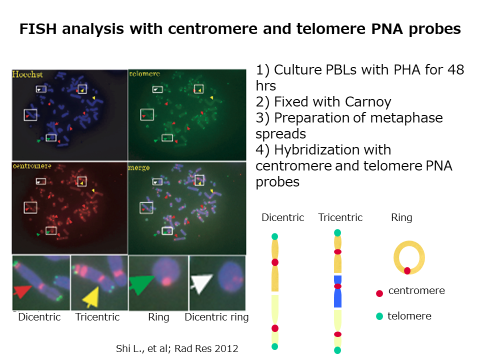
Next, we investigated the effects of CT examinations. We examined the chromosomal aberrations in blood cells before and after the CT scan. As a result, we could show the increase of chromosome aberrations after the CT scan.
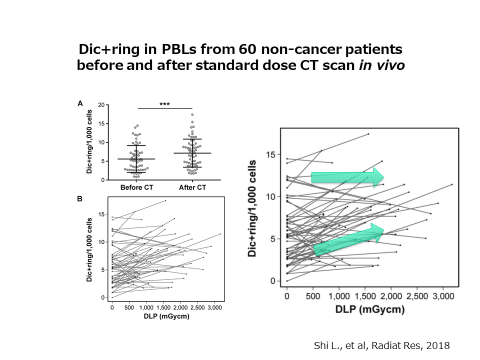
To our interest, the study also revealed the presence of individual differences in the increment of abnormal chromosomes induced by the radiation exposure, even at the same effective dose. We confirmed the individual difference of radiation sensitivity against low dose irradiation in healthy volunteers. While the numbers of chromosome aberrations from some volunteers showed a dose-dependent linear increase (left), those from others did not (right). We are now trying to find out the reason for individual differences in the chromosomal sensitivity to low-dose exposure.
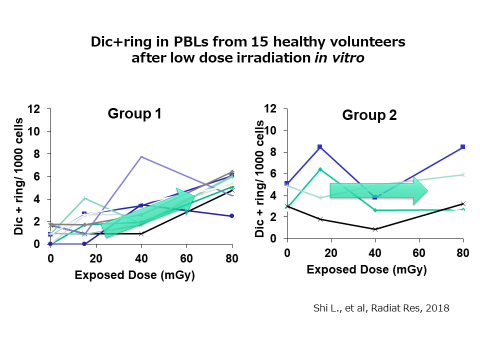
Recent advances in CT technology have made it possible to take clear images even with lower-dose CT examinations. Lung cancer screening for high-risk people using such low-dose CT examinations is in the spotlight because it is reported to reduce the mortality rate by about 20%. On the other hand, the extent of health effects of such low-dose CT examinations is under discussion.
Therefore, we examined the increase of chromosome aberrations induced by low-dose CT examinations. This time, we investigated not only the chromosome aberrations but also the phosphorylation of H2AX. H2AX is a histone protein which is to be chemically modified, phosphorylation, when chromosomal DNA is damaged. Phosphorylated histone H2AX (γH2AX) gathers at the site of damaged DNA to form a nuclear domain called γH2AX focus. Since one γH2AX focus contains one DNA damage, we can detect chromosomal DNA damage quite sensitively by counting the number of γH2AX focus with a microscope.
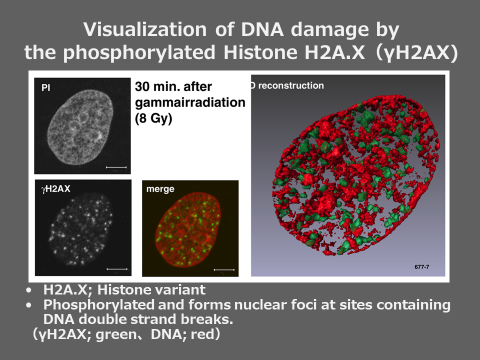
Using the samples from the outpatients of Hiroshima University Hospital who underwent low-dose CT (LD-CT) and standard-dose CT (SD-CT) examinations, we compared the number of γH2AX focus and chromosome aberrations before and after CT examinations. The number of γH2AX focus and chromosome aberrations increased after a single SD-CT examination, indicating that both methods are sensitive enough to clearly detect the biological effect of SD-CT. On the other hand, there was no differences in them after a single LD-CT examination. The result showed that LD-CT examinations damaged the chromosomal DNA only to the extent that could not be found with these methods. Since the screening by LD-CT for high-risk lung cancer is an effective test that reduces mortality by as much as 20%, this finding supports the justification of LD-CT examination in lung cancer screening. This study was published in “Radiology,” a journal of radiology in the United States, in March 2020.
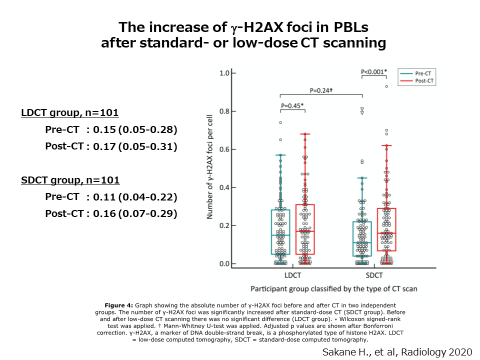
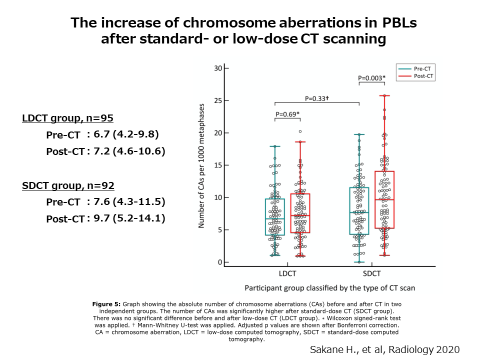
We are trying to develop more sensitive and high-speed measurements to detect the biological effects of radiation exposure such as genome damage, for the establishment of "next-generation radiology" that makes use of medical radiation more effectively and safely.